The most complex part of the human body just got a whole lot simpler. Recent breakthroughs in scientific research have allowed scientists to map the brain at a single cell resolution.
The brain is the most important organ in the human body, driving our thoughts, ambitions, creativity, and uniqueness. Not surprisingly, the brain is also the most complex and least understood organ. Until recently, scientists were not able to distinguish the molecular blueprint of individual brain cells, but with ‘next generation single cell sequencing’ they are beginning to define the molecular signatures of cells in our brains and discover unique cell types and biological pathways. As a result, research has been able to determine the largest map of the human brain thus far.
The previous technology of ‘bulk sequencing’ is unable to uncover mutations or other molecular alterations within individual cells. This not only presented an issue to scientists attempting to better understand the innerworkings of the brain, but also to clinical practitioners. Mutations in single cells can make some tumors resistant to cancer treatment. With next generation single cell sequencing, pathologists looking at samples of tumors or cancerous tissues can prescribe more specific and accurate therapies for cancer patients.
Most significantly, with the ability to detect and analyze individual components of tumors and brain tissues, cancer scientists achieve a deeper understanding of how cancer affects the brain on a cellular level. Single cell sequencing allows researchers to determine what part of their genetic code tumors use to make themselves invisible to immune cells. Scientists are able to track mutations or other molecular alterations that occur in individual cells and prevent the emergence of tumors that are immune to treatment.
How Single Cell Sequencing Works
Single cell sequencing measures the unique DNA or mRNA of cells, one by one. mRNA or “messenger RNA” is a molecule that instructs the cells to function and create proteins in a specific way. They carry information from a cell’s DNA, which stores an organism’s genetic code, and can be altered (or mutated) in cancer.
One approach to single cell sequencing is to separate cells and then individually assign them an unique barcode. This code serves to differentiate the mRNA of the tagged cell with the mRNA of neighboring cells. Then the scientists sequence the mRNA from each unique cell and use mathematical algorithms to analyze how these cells are similar and different from one another.
Another method is to split cells into groups and assign a barcode to the mRNA in a specific group. When the cells disperse and are once again collected into different groups, each cell in that following distribution will have a unique tag in its mRNA. This allows the creation of unique barcodes that differentiate the cells without the work needed to individually split them up.
Single Cell Sequencing in Action
Dr. Susana Ramos is a postdoctoral fellow in Dr. Nadejda Tsankova’s research laboratory in the Departments of Pathology and Neuroscience at the Icahn School of Medicine at Mount Sinai. There, her project is to utilize single cell sequencing to study the development of the human brain during the third trimester. The lab’s breakthrough study, An atlas of late prenatal human neurodevelopment resolved by single-nucleus transcriptomics, was published in Nature Communications, one of the most prestigious journals in the scientific community.
The brain expands exponentially during the third trimester, and during this time develops glial cells. This process, known as gliogenesis, is when stem cells in the brain become different types of glial progenitor cells. These cells later become astrocytes or oligodendrocytes, which support the brain’s neurons by providing them nutrients and insulation, and clearing out debris.
“Through sequencing, we order cells by how they relate to each other developmentally, meaning you can infer mathematically which immature cell gave rise to its mature prodigy (mother-daughter cells),” said Ramos. During development, humans are first made up of stem cells that later become complex and unique, becoming neurons, skin cells, liver cells, and more. “As you age, your cells get more and more differentiated and subspecialized,” said Ramos.
Single cell sequencing allowed Ramos to observe what cell type in the developmental period gave rise to what adult cell, and to determine the similarities and differences between the two. “You can see rare cell types that we didn’t even know existed,” said Ramos.
By analyzing samples, the lab discovered a rare glial Intermediate Progenitor Cell (gIPC) that exists in the stage between stem cells and mature glial cells (astrocytes or oligodendrocytes). These glial progenitor cells resemble the genetic makeup of brain tumors in adults, even though gIPCs are mostly found during the third trimester of development.
In order to observe these interesting similarities, Ramos utilized the method of single cell sequencing that individually separates each cell. “What we do to sequence the cells is we analyze the mRNA and then add the barcode, which is a unique sequence so when you read the mRNA you know what cell it belongs to,” said Ramos.
After birth the gIPC progenitor cells that the lab discovered slowly disappear, becoming either mature astrocytes or oligodendrocytes. However, because the cells strikingly parallel brain tumors, Ramos continues to study what occurs during gliogenesis to uncover the mysteries behind how cancer grows.
“We don’t know which cells start as the tumor cells, but we think that they revert back to the progenitor state,” said Ramos. “Glial progenitors become astrocytes, so it may be possible to revert tumors to astrocytes.”
To research this phenomenon, Ramos experiments on the brains of mice. “We insert human tumors into a mouse brain, recreating what we see in the patient,” said Ramos.
All the information gathered through single cell sequencing is then analyzed through code, producing the data analysis which determines these cell types relate to each other. “I seem to have become a computational biologist,” said Ramos.
Single Cell Sequencing and Cancer Research
Being able to track the individual movement of cells has led to immense breakthroughs in cancer research. Dr. Balagopal Pai, another postdoctoral fellow in Dr. Tsankova’s lab that contributed to the study on glial progenitors, continues to utilize single cell sequencing in studies regarding brain tumors.
Brain tumors often mess up more than just one particular area, threatening multiple different cell types. In order to give accurate diagnosis and propose the most successful method of removing the tumor, it becomes vital to determine exactly which types of cells are targeted and which genes are active in the affected area.
When analyzing the biology of the tumor as a whole, scientists could only understand a broad summary of the genes and activity of different regions within the brain. “You wouldn’t know which specific cell type is being messed up by the tumor,” said Pai.
Now with single cell sequencing, scientists can study the biology of each cell within the tumor and determine which are the most infiltrative and the most resistant to therapy. This technology allows scientists to study the normal cell types next to the tumor and understand their interactions with the tumor.
What Pai now researches involves tagging individual cells with different codes to determine what gene comes from what particular cell. “When I initially started doing research, I was working with many other topics,” said Pai. “When I started working with cancer, I realized that single cell sequencing was more important for cancer research rather than any other study.”
Cell Analysis in Action
When I went to interview the members of the Department of Pathology at Mount Sinai, the lab was particularly active.
Whenever a patient undergoes surgery to remove a tumor, there is a limited amount of time to secure the tissue, diagnose the tumor, and preserve the specimen. The day that I arrived, a young patient with a particularly complicated tumor had undergone surgery that morning.
I rushed through Mount Sinai’s maze of underground tunnels with the Director of Neuropathology, Dr. Tsankova, and Dr. Thenzing Silva. In a room full of imposing scientific machines, Dr. Tsankova sliced the tissue and preserved it in a special buffer called pipes solution on ice.
“When there’s a valuable tissue, I distribute parts to everyone who needs a part for their research or clinical use,” said Dr. Tsankova. That specific tissue was in particularly high demand because it came from a patient with a rare tumor whose origins and treatments are unknown and infrequently studied.
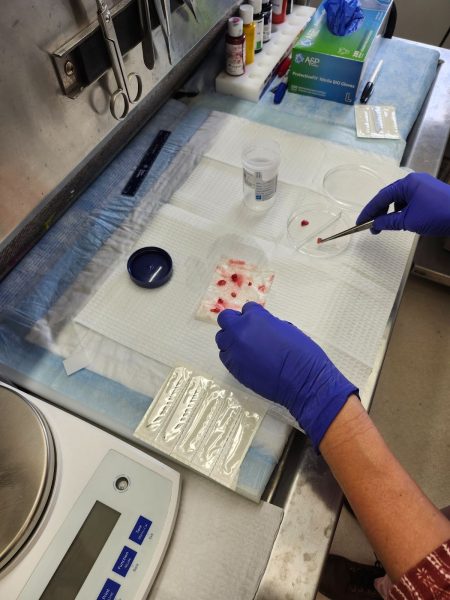
To determine what the cells in the tumor represented, Dr. Tsankova squashed a tiny slice of the tissue on a slide and dipped in a dozen solutions to stain the cells. Under the microscope, the sample was stained purple, with the tumor cells appearing as large purple plums compared to smaller, grape sized cells.
Single cell analysis can even be performed on a sample under a microscope, allowing pathologists to analyze cells promptly, performing rapid and accurate diagnosis. “We can take the stained tissue on the slide and automatically apply the barcode to that issue. Then we can see which region is enriched with neurons or what genes are expressed in any particular spot,” said Pai.
As both technological and scientific advancement continues, the vast mysteries of the human brain become at the same time clearer and more complex. Each enlightening discovery begets twice as many questions, advancing both human knowledge and medical applications.
Single cell sequencing research performed by many different labs at Mount Sinai and at numerous other institutions provides a novel and high-resolution look at development and tumor biology, bringing us one step closer to finding more extensive treatments for brain cancers.
“You can see rare cell types that we didn’t even know existed,” said Dr. Susana Ramos, a postdoctoral fellow in Dr. Nadejda Tsankova’s research laboratory in the Departments of Pathology and Neuroscience at the Icahn School of Medicine at Mount Sinai.